Early evolution of the Earth - The origin of the planet Earth
Recent earthquakes caused by displacements of the earth's crust, together with lava from the eruption of active volcanoes, represent only the latest in a long series of events through which our planet has reached its present form and structure. The geological processes that occur within the Earth can be better understood when observed in the context of much earlier events in the history of the Earth.
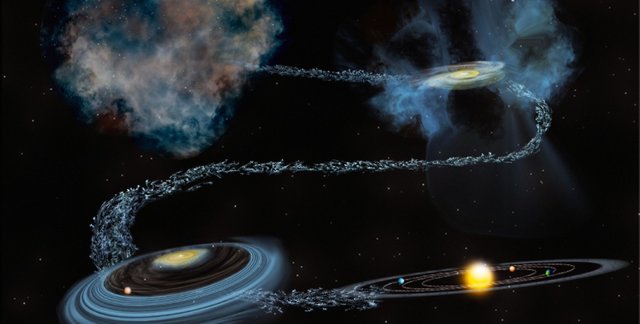
The origin of the planet Earth
The following scenario describes the most widely accepted opinions about the origin of our Solar System. Although this model is presented as a fact, remember that like all scientific hypotheses, it is subject to revision and even exposed to absolute rejection. However, it continues to be the most coherent set of ideas to explain what we observe today. Our scenario begins about 12,000 to 15,000 million years ago with the Big Bang, an incomprehensibly large explosion that sent out all the matter of the universe at incredible speeds. At that time, the remains of the explosion, which consisted almost entirely of hydrogen and helium, began to cool and condense into the first stars and galaxies.
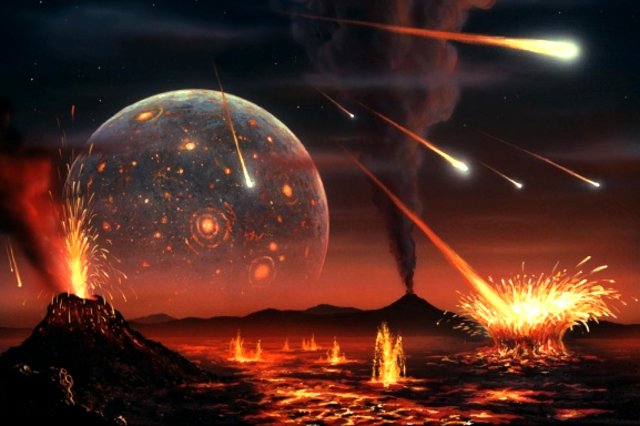
In one of these galaxies, the Milky Way, was where our Solar System and planet Earth took shape. Earth is one of the nine planets that, along with about a dozen moons and numerous smaller bodies, revolve around the Sun. The orderly nature of our Solar System leads most researchers to infer that Earth and the others planets were formed essentially at the same time, and from the same primordial matter, as the Sun. The primitive nebula hypothesis suggests that the bodies of our Solar System were formed from a huge, rotating cloud called the solar nebula. Hydrogen and helium atoms generated during the Big Bang, microscopic dust grains and matter expelled from long-dead stars formed the solar nebula.
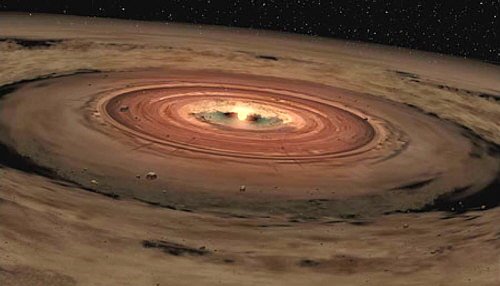
(Nuclear fusion in stars converts hydrogen and helium into the other elements found in the universe.) About 5,000 million years ago, this immense cloud of gases and tiny grains of heavier elements began to slowly contract due to the gravitational interactions between its particles. An external influence, such as a shock wave from a catastrophic explosion (supernova), could have caused the collapse. When it collapsed, this slowly spinning, spiraling cloud rotated faster and faster for the same reason that an ice skater does it when he throws his arms around himself. In the end, the gravitational attraction was balanced by the centrifugal force caused by the rotational movement of the cloud. But this time, the cloud, before extensive, had taken the form of a flat disk with a large concentration of material in the center called protosol (Sun in formation).
(Astronomers are fairly certain that the nebula formed a disk because similar structures have been detected around other stars.) During the collapse, gravitational energy was converted to thermal energy (heat), which caused the interior temperature of the nebula will increase dramatically. At these high temperatures, the dust grains decomposed into molecules and atomic particles. However, at distances after the orbit of Mars, temperatures probably remained quite low. At 200 ° C, it is possible that the small particles on the outside of the nebula were covered by a thick layer of ice consisting of water, carbon dioxide, ammonia and methane frozen. (Some of this material still resides in the confines of the Solar System, in the region called the Oort cloud.) The disk-shaped cloud also contained considerable amounts of lighter gases: hydrogen and helium. The formation of the Sun marked the end of the period of contraction and, therefore, the end of gravitational heating. The temperatures of the region where the inner planets are now began to diminish. This decrease in temperature caused substances with high melting points to condense into small particles that began to coalesce. Materials such as iron and nickel and elements that make up the minerals that make up rocks (silicon, calcium, sodium, etc.) formed metal and rock masses that orbit around the Sun. Repeated collisions caused the union of these masses in larger bodies, the size of an asteroid, called protoplanets. , which in a few tens of millions of years grew to become the four inner planets we call Mercury, Venus, Earth and Mars, not all of these masses of matter were incorporated into the protoplanets.
The rocky and metallic pieces that remained in orbit are called meteorites when they survive an impact with the Earth. As the protoplanets attracted more and more material, the high speed impact of the remains of the nebula caused the temperature rise of these bodies. Because of their relatively high temperatures and weak gravitational fields, the inner planets could not accumulate many of the lighter components of the nebula. The lightest of these components, hydrogen and helium, were finally swept from the inner part of the Solar System by the solar winds.
At the same time as the inner planets were being formed, the larger outer planets (Jupiter, Saturn, Uranus, and Neptune) were also being developed, along with their extensive satellite systems. Because of the low temperatures due to the long distance of the Sun, the material from which these planets formed contained a high percentage of ice (water, carbon dioxide, ammonia and methane) and rocky and metallic debris. The accumulation of ice explains in part the large dimensions and low density of the outer planets. The two planets with the largest mass, Jupiter and Saturn, had a sufficient surface gravity to attract and sustain large quantities of the lighter elements, hydrogen and helium.
Formation of the structure in layers of the Earth
As the material accumulated to form the Earth (and shortly thereafter), the high-speed impact of the remains of the nebula and the disintegration of the radioactive elements caused a constant increase in the temperature of our planet. During this period of intense warming, the Earth reached sufficient temperature for the iron and nickel to begin to melt. The fusion produced drops of heavy metal that penetrated towards the center of the planet. This process happened quickly on the geologic time scale and formed the dense, iron-rich core of the Earth. The first heating period triggered another process of chemical differentiation, whereby the melt formed floating masses of molten rock that rose to the surface, where they solidified and formed the primitive crust. These rocky materials were enriched with oxygen and "lithophilic" elements, especially silicon and aluminum, with lower amounts of calcium, sodium, potassium, iron and magnesium. In addition, some heavy metals such as gold, lead and uranium, which have low melting points or were very soluble in the rising melts, were removed from the interior of the Earth and concentrated in the developing crust. This first period of chemical segregation established the three basic divisions of the interior of the Earth: the iron-rich core; the primitive bark, very thin; and the thickest layer of the earth, called the mantle, that lies between the core and the crust. An important consequence of this period of chemical differentiation is that it allowed large quantities of gaseous compounds to escape from the interior of the Earth, as is currently the case during volcanic eruptions.
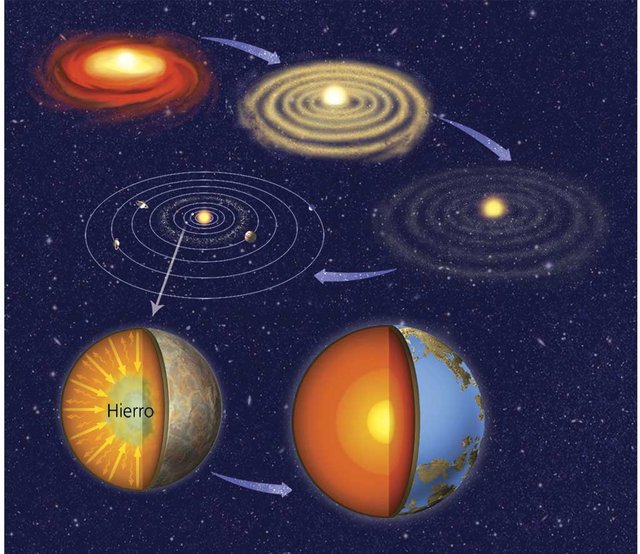
Thanks to this process, the primitive atmosphere gradually evolved. It was on this planet, with that atmosphere, where life appeared as we know it. After the events that established the basic structure of the Earth, the primitive crust was lost due to erosion and other geological processes, so that we do not have any direct record of its composition. When and how exactly the continental crust appeared (and with it the first terrestrial continental masses) is a question that is still under investigation. However, there is general agreement that the continental crust was formed in a gradual manner during the last 4 billion years. (The oldest rocks discovered to date are isolated fragments, found in northwestern Canada, which have radiometric dates of about 4,000 million years.), The Earth is an evolving planet whose continents (and ocean basins) have constantly changed of form and even situation during a large part of this period.
Internal structure of the Earth
In the previous section, you learned that the segregation of material that started very early in Earth's history resulted in the formation of three layers defined by their chemical composition: the crust, the mantle and the core. In addition to these three layers of different composition, the Earth can be divided into layers based on its physical properties. The physical properties used to define these zones are their solid or liquid character and how ductile or resistant they are. The knowledge of both types of structures in layers is essential for the understanding of basic geological processes, such as volcanism, earthquakes and mountain formation.
Layers defined by their composition
Cortex.
The crust, the comparatively thin external rocky layer of the Earth, is generally divided into oceanic crust and continental crust. The oceanic crust is about 7 kilometers thick and is composed of dark igneous rocks called basalts. On the contrary, the continental crust has an average thickness of between 35 and 40 kilometers, but it can exceed 70 kilometers in some mountainous regions. Unlike the oceanic crust, which has a relatively homogeneous chemical composition, the continental crust consists of many types of rocks. The upper level of the continental crust has the average composition of a granitic rock called granodiorite, while the composition of the lower part of the continental crust is more similar to basalt. The continental rocks have an average density of about 2.7 g / cm3 and some have been discovered whose age exceeds 4,000 million years. The rocks of the oceanic crust are younger (180 million years or less) and denser (about 3.0 g / cm3) than the continental rocks.
Mantle.
More than 82 percent of the Earth's volume is contained in the mantle, a solid rock covering that extends to a depth of 2,900 kilometers. The boundary between the crust and the mantle represents a change in chemical composition. The type of dominant rock in the upper part of the mantle is the peridotite, which has a density of 3.3 g / cm3. At a greater depth, the peridotite changes and adopts a more compact crystalline structure and, therefore, a higher density. Core. It is believed that the composition of the core is an alloy of iron and nickel with smaller amounts of oxygen, silicon and sulfur, elements that easily form compounds with iron. At the extreme pressure of the core, this iron-rich material has an average density of about 11 g / cm3 and approaches 14 times the density of water in the center of the Earth.
How do we know what we know?
At this point you should ask yourself: How did we know the composition and structure of the interior of the Earth? You can assume that samples have been taken from the interior of the Earth directly. However, the world's deepest mine (the Western Deep Levels mine in South Africa) has a depth of only 4 kilometers, and the deepest drilling in the world (completed in the Kola peninsula, in Russia, in 1992) only it penetrates approximately 12 kilometers. In essence, humans have never drilled a hole in the mantle (and never will in the core) in order to draw direct samples of these materials. Despite these limitations, theories have been developed that describe the nature of the interior of the Earth and that coincide with most of the data from the observations. Thus, our model of the interior of the Earth represents the best deductions we can make based on the available data.
For example, the layered structure of the Earth has been established by indirect observations. Each time an earthquake occurs, energy waves (called seismic waves) penetrate the interior of the Earth, in a way similar to how X-rays penetrate the human body. Seismic waves change speed and deviate and reflect when crossing areas with different properties. An extensive set of control stations around the world detects and records this energy. With the help of computers, these data are analyzed, which are then used to determine the structure of the Earth's interior. In Chapter 12, "Inside the Earth," you will find more information on how this is done. What evidence do we have to support the supposed composition of the interior of our planet? It may be surprising to know that rocks that originated in the mantle have been collected on the surface of the Earth, including samples containing diamonds, which, according to laboratory studies, can only be formed in environments with high pressure. Since these rocks must have crystallized at depths greater than 200 kilometers, it is deduced that they are samples of the mantle that suffered very little alteration during their ascent to the surface.
In addition, we have been able to examine the layers of the upper mantle and the oceanic crust covering it that have been pushed above sea level in places like Cyprus, Newfoundland and Oman. Establishing the composition of the nucleus is another completely different matter. Due to its great depth and high density, no core sample has reached the surface. However, we have significant evidence to suggest that this layer consists mainly of iron. Surprisingly, meteorites provide important clues about the composition of the core and mantle. (Meteorites are solid extraterrestrial objects that hit the surface of the Earth.) Most meteorites are fragments derived from collisions of larger bodies, mainly from the asteroid belt located between the orbits of Mars and Jupiter. They are important because they represent samples of the material (planetesimals) from which the inner planets were formed, including the Earth. Meteorites are mainly composed of an alloy of iron and nickel (metal), silicate minerals (rock) or a combination of both materials (mixed). The average composition of rocky meteorites is very similar to what the mantle is supposed to have.
On the other hand, metal meteorites contain a much higher percentage of this metal material than that found in the earth's crust or mantle. If, in fact, the Earth was formed from the same material in the solar nebula that generated the meteorites and other inner planets, it must contain a much higher percentage of iron than is found in the rocks of the crust. Therefore, we can conclude that the nucleus is enormously rich in this heavy material. This point of view is also supported by studies of the composition of the Sun, which indicate that iron is the most abundant substance found in the Solar System that has the density calculated for the nucleus. In addition, the Earth's magnetic field requires that the nucleus be made of an electrically conductive material, such as iron. Since all available evidence suggests that a large part of the core is composed of iron, we take this as fact, at least until new evidence tells us otherwise.
Bibliography :
- https://en.wikipedia.org/wiki/History_of_Earth
- http://www.uh.edu/~geos6g/1376/originearth6.html
- https://www.space.com/19175-how-was-earth-formed.html
- https://link.springer.com/article/10.1007/BF03043789
- http://www.geosci.usyd.edu.au/research/re_early.shtml
- https://www.annualreviews.org/doi/abs/10.1146/annurev.ea.18.050190.001533?journalCode=earth
You received a 10.0% upvote since you are not yet a member of geopolis and wrote in the category of "geology".
To read more about us and what we do, click here.
https://steemit.com/geopolis/@geopolis/geopolis-the-community-for-global-sciences-update-4
Nice article.
You made a small typo in your second paragraph. The age of the universe should be given in billion years.